Applied Nuclear Technology
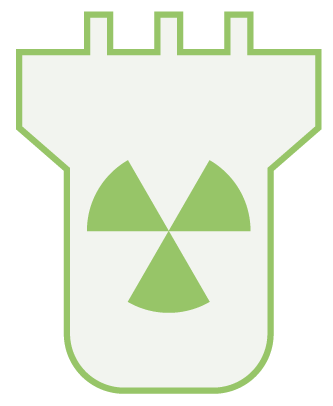
The foundation is a leader in modeling small modular nuclear reactors (SMRs), which represent a significant shift towards more manageable, economically feasible nuclear power solutions. Our models help optimize the design and operation of SMRs, focusing on improving safety features, reducing waste, and enhancing scalability. This research is pivotal as SMRs hold the potential to spread nuclear technology to areas not serviced by traditional large-scale reactors, offering a cleaner energy alternative.
Our sophisticated simulations delve into the thermal-hydraulic performance of SMRs, ensuring that these systems can achieve maximum efficiency while maintaining strict safety standards. We also explore modular design approaches that allow for reactors to be built in a factory setting and assembled onsite, significantly reducing construction costs and timelines. This modular strategy not only accelerates the deployment of nuclear power but also allows for greater flexibility in energy planning and management across Europe.
The environmental impact of SMRs is another critical area of our research. By modeling the entire lifecycle of these reactors, from construction through decommissioning, we aim to minimize their carbon footprint and enhance their sustainability. This comprehensive analysis helps in developing strategies that align with global environmental standards and support the European Green Deal, positioning nuclear energy as a key player in the transition to a low-carbon economy.
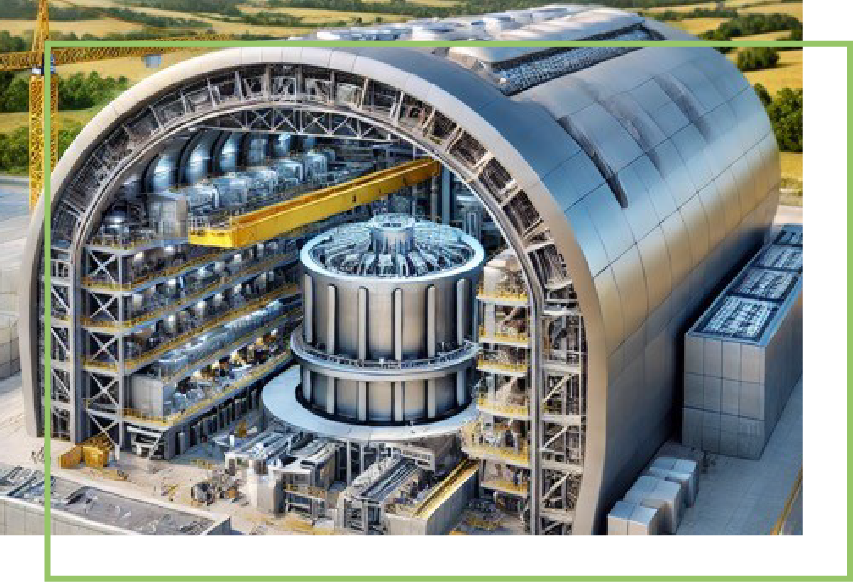
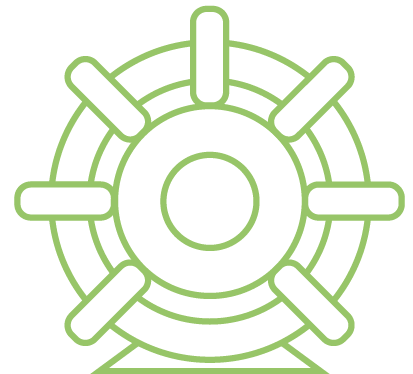
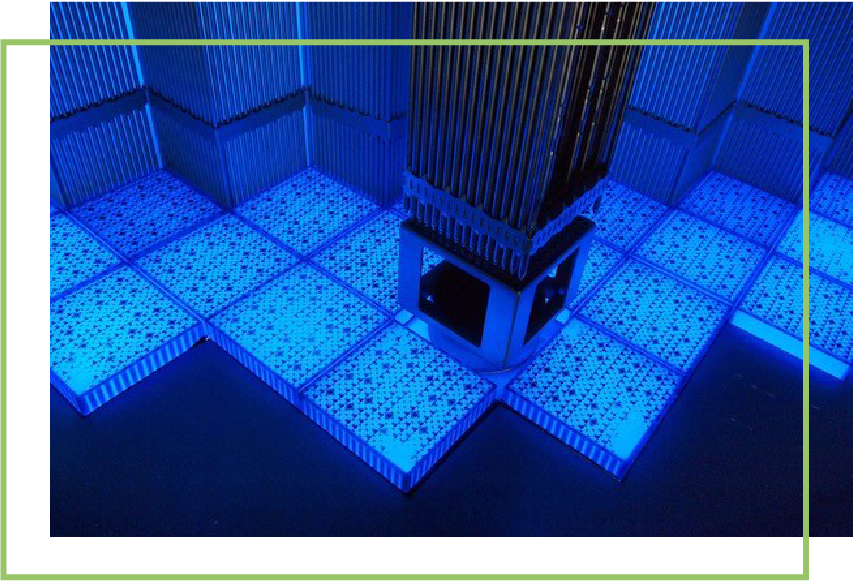
Our work with accelerator-driven nuclear systems (ADS) focuses on harnessing these technologies for waste reduction and improved safety. ADS uses particle accelerators to induce nuclear reactions, offering a controlled environment that minimizes the risk of meltdowns. Our advanced techniques evaluate the dynamics and feasibility of these systems, paving the way for their practical application in managing nuclear waste and producing energy.
The potential of ADS to transmute long-lived radioactive isotopes into shorter-lived ones is particularly transformative. This capability could solve some of the most persistent challenges in nuclear waste management, reducing the radiotoxicity and volume of waste that needs to be stored or disposed of. Our foundation is at the forefront of developing scalable ADS models that can be implemented in national nuclear waste management strategies, offering a path to safer and more sustainable nuclear energy production.
Additionally, the use of ADS in energy production is explored through our models investigating particle beam dynamics and neutron flux distribution within a reactor. These models help optimize the design of ADS for higher energy output and stability, ensuring that they can be a viable alternative to conventional nuclear reactors. Our research not only advances the technical feasibility of ADS but also contributes to policy discussions on nuclear energy’s role in achieving energy security and sustainability goals.
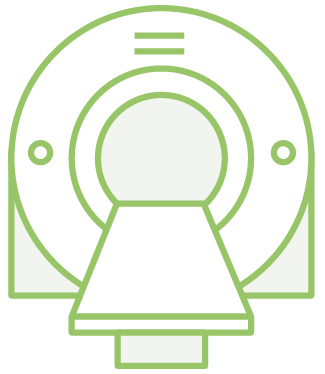
Our commitment lies in revolutionizing nuclear medicine through advanced experimental research, theoretical models, and innovative design. By integrating cutting-edge experimental methodologies and sophisticated theoretical frameworks, we aim to enhance radiotherapy treatments, including highly targeted therapies such as boron neutron capture and proton accelerator therapies. Our approach allows us to predict the behavior of radioactive particles in the human body, optimize dosages, and minimize exposure to healthy tissues.
Our research significantly improves treatment efficacy for cancer and other diseases. Through rigorous experimental studies and refined theoretical analysis, we determine the precise trajectories of neutron and proton beams, ensuring they converge accurately at the tumor site. This targeted strategy not only reduces damage to surrounding healthy tissues—a common challenge in traditional radiotherapy—but also boosts the probability of successful outcomes.
In parallel with treatment planning, our work supports the development of novel radiopharmaceuticals. These advanced drugs are designed to transport radioactive isotopes directly to specific cell types, such as cancer cells, by leveraging our deep understanding of molecular interactions and cellular targets. This research not only expands our knowledge of cellular mechanisms but also sets the stage for next-generation targeted therapies, promising treatments that are both highly effective and minimally invasive.
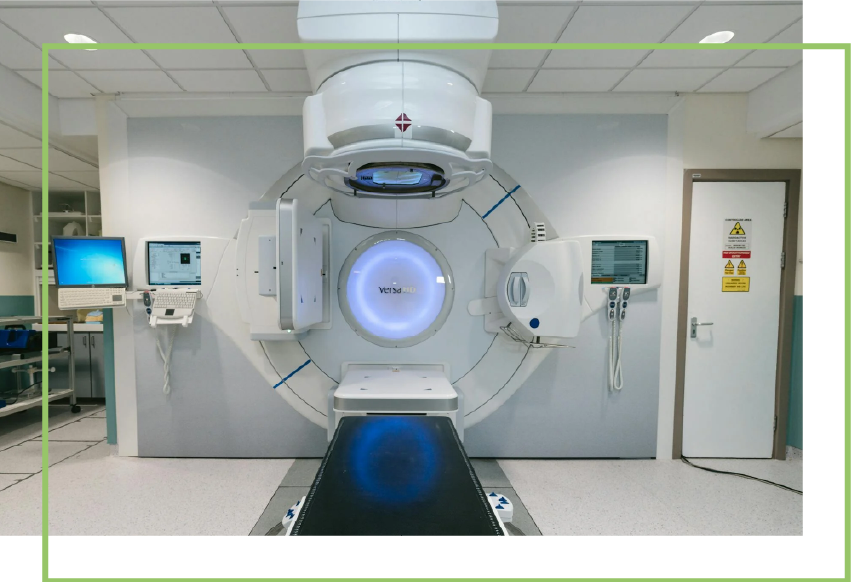