Virtual and Modeling Lab
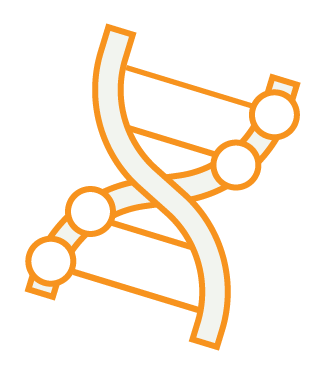
Our work in advanced molecular modeling is key to unlocking new therapeutic possibilities. Using state-of-the-art computational techniques, we simulate and analyze the interactions between pharmaceutical compounds and biological targets to understand their efficacy and potential side effects. This predictive modeling is vital in speeding up the drug development process, allowing us to sift through thousands of molecular candidates and focus on those with the most promise for treating diseases at their root cause.
Moreover, our researchers use molecular modeling to study the structure and function of proteins involved in critical biological processes and diseases. By understanding these macromolecules at a molecular level, we can identify unique intervention points for new drugs, leading to more effective treatments with targeted action. This not only enhances our understanding of complex diseases but also supports the development of novel therapies that can disrupt disease progression.
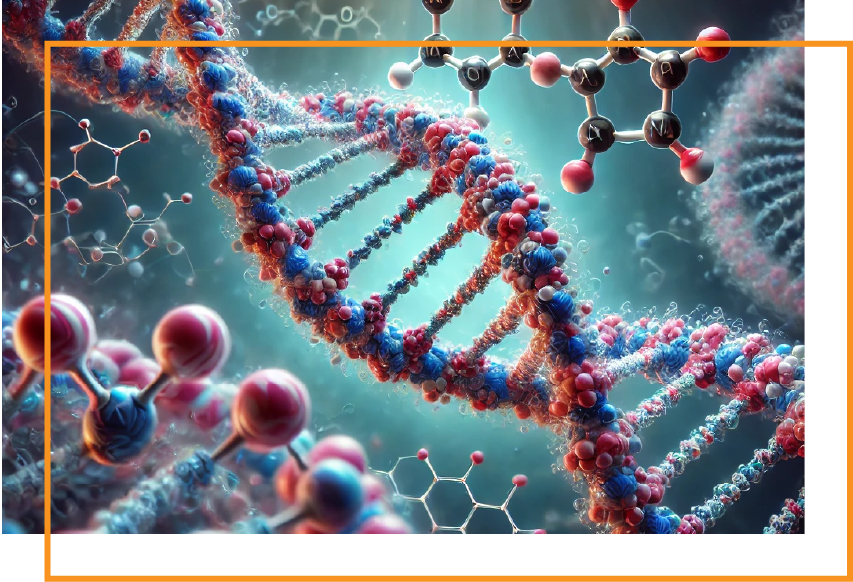
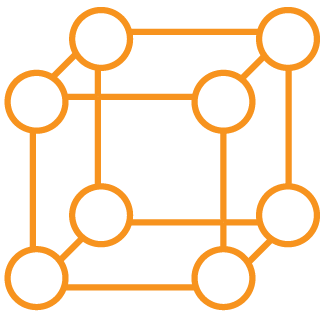
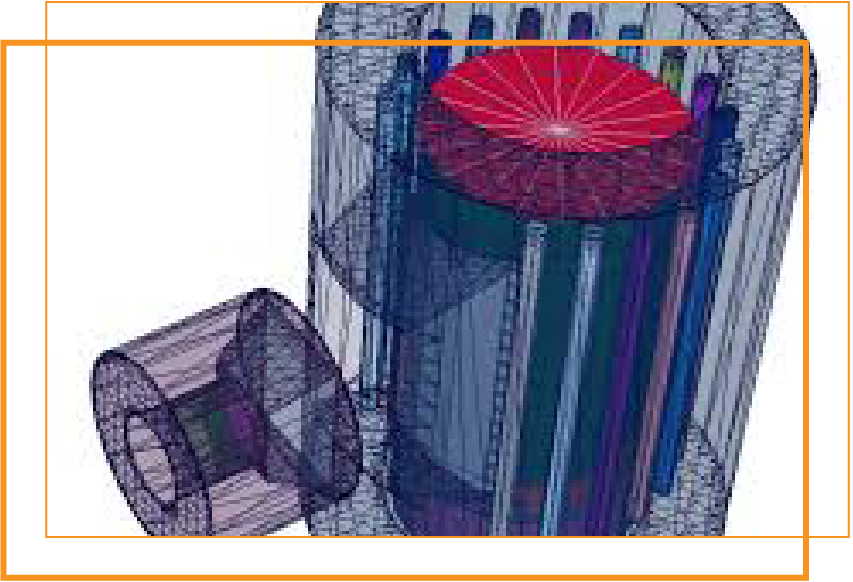
At the core of our material science research is the use of Density Functional Theory (DFT) and Large-scale Atomic/Molecular Massively Parallel Simulator (LAMMPS) codes. These tools allow us to simulate and analyze the atomic and molecular interactions of materials used in nuclear settings. This modeling is crucial for developing new materials that can perform under the harsh conditions of nuclear reactors and medical applications, leading to innovations in safety and functionality.
DFT and LAMMPS provide the computational power necessary to handle the complex quantum mechanical behaviors of materials at an atomic level. This capability is vital for designing materials that are not only radiation resistant but also capable of self-healing and high thermal conductivity. Our ongoing projects leverage these codes to explore novel ceramic composites and metallic alloys that could redefine the core materials used in nuclear fission and fusion reactors.
Furthermore, our team uses these advanced computational methods to investigate degradation mechanisms like corrosion and creep under nuclear reactor conditions. Understanding these processes at a molecular level aids in developing more robust materials that maintain their integrity over longer operational lifespans, reducing the frequency and costs of maintenance. These advancements are critical for the sustainability and safety of nuclear energy systems, providing a deeper understanding that guides both material selection and reactor design.
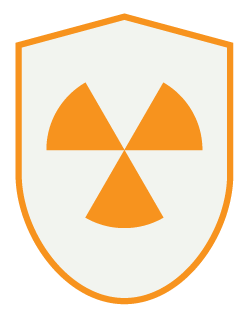
Our research in radiation-resistant materials is essential for the development of safer and more durable nuclear environments. Utilizing sophisticated modeling techniques, we aim to design materials that can withstand extreme conditions in nuclear reactors and other radioactive settings. By predicting how materials behave under radiation, our work supports the longevity and reliability of nuclear systems, reducing risks and enhancing performance.
In our pursuit to enhance the radiation resistance of materials, we employ multi-scale simulation frameworks that bridge quantum mechanical calculations and macroscopic phenomena. This approach allows for a deeper understanding of the fundamental mechanisms that govern material behavior under high radiation, aiding in the identification and synthesis of novel materials that offer superior performance. Our collaborations with international laboratories and industries ensure that these materials are not only theoretical models but also viable for real-world application in reactors, space crafts, and medical facilities.
Moreover, our team is actively involved in the development of coatings and alloys that could redefine norms for radiation shielding. Experimentation with nanostructured materials and composite layers offers promising avenues for creating thinner, lighter, and more effective shielding solutions than currently exist. These advancements have profound implications for the safety of both personnel and equipment in high-radiation environments such as space exploration and radiotherapy.
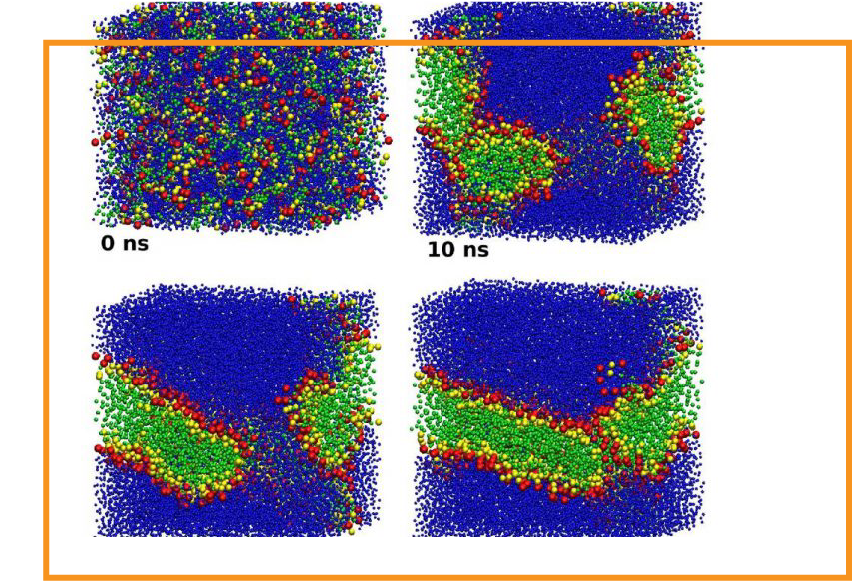
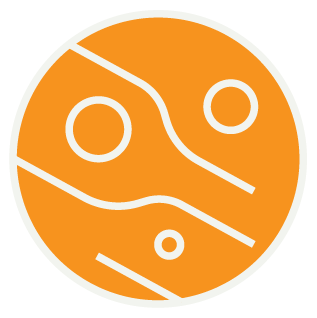
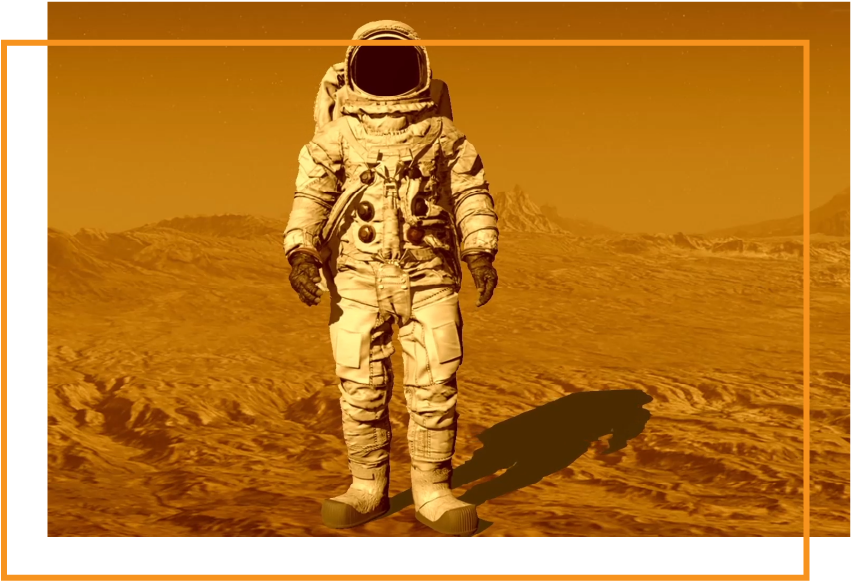
Our foundation is pioneering the development of materials designed to protect against the harsh radiation environments encountered in space and on Mars. Through advanced computational modeling, we identify and design materials that can withstand the intense cosmic rays and solar radiation that pose significant risks to missions beyond Earth’s atmosphere. Our focus extends to shielding for pacecraft, habitats, and space suits, ensuring that astronauts are protected as they explore and potentially colonize other planets. Utilizing simulation tools such as Monte Carlo methods and particle transport codes, we model the interaction between radiation and various shielding materials. These simulations help us understand how high-energy particles penetrate and interact with different substances, guiding us in the development of composites and alloys specifically engineered for space applications. Our research is critical for long-duration missions, where traditional materials may fail to offer sufficient protection from the cumulative effects of space radiation.
In collaboration with international space agencies, our efforts are not only theoretical but also practical. We test our materials in simulated space conditions, including exposure to vacuum and extreme temperatures, to ensure they can endure the rigors of space travel and Martian environments. This research is essential for the safety and success of future manned missions to Mars and other celestial bodies, aiming to create sustainable and safe living conditions for astronauts far from Earth.
These three paragraphs expand the webpage text to include the crucial aspect of developing materials for radiation protection in space and on Mars, showcasing the foundation’s role in supporting deep space exploration and colonization.